The History of Hydrogen Discovery and Development
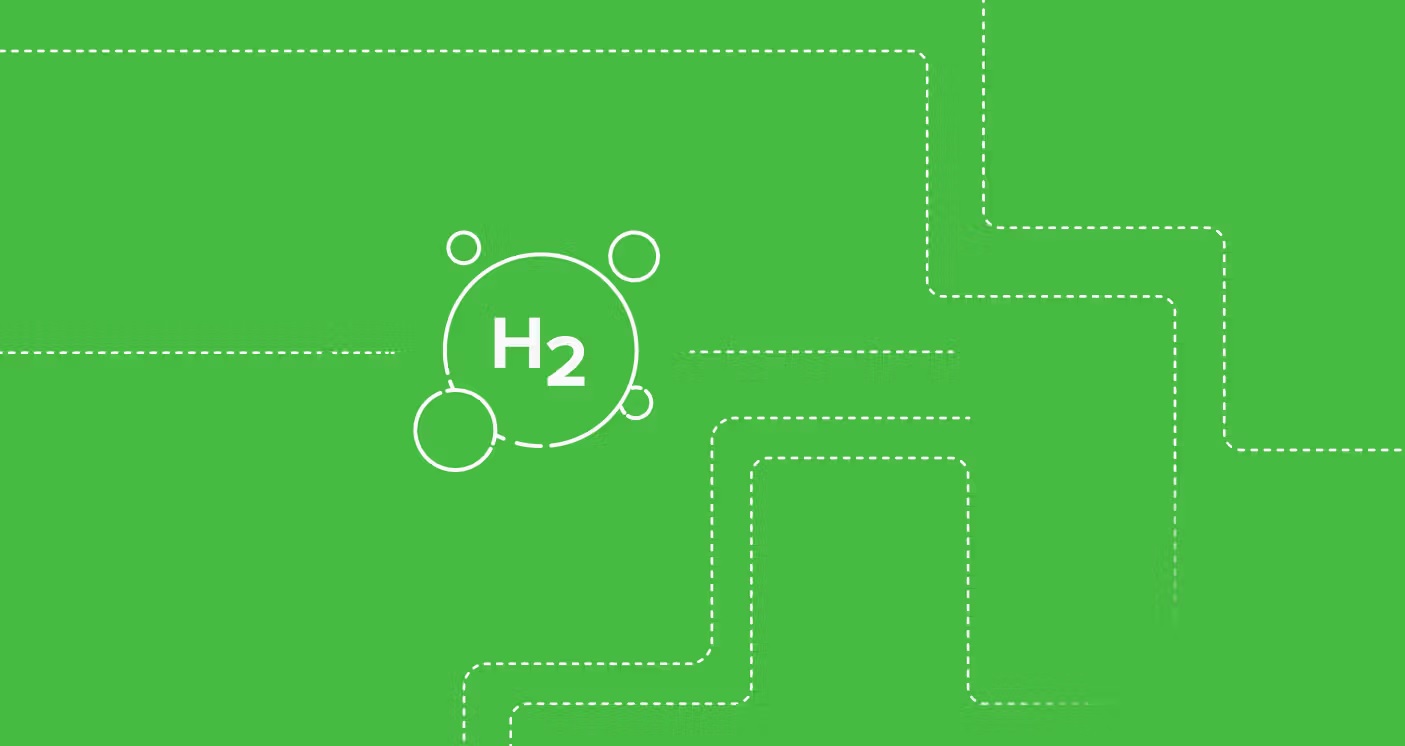
Introduction
Hydrogen, the most abundant element in the universe, has played a crucial role in scientific advancements and industrial applications. From its initial discovery in the 18th century to its modern use in energy and aerospace, hydrogen has transformed various industries and continues to be at the forefront of clean energy solutions.
The Discovery of Hydrogen
Henry Cavendish and the Identification of Hydrogen (1766)
In 1766, British scientist Henry Cavendish was the first to recognize hydrogen as a distinct gas. Using controlled experiments, he demonstrated that when metals such as zinc and iron reacted with acids, they released an inflammable gas. Cavendish further identified that when burned, this gas produced water, leading to its initial name: "inflammable air."
Recent analyses of Cavendish's work suggest that his precise measurements, using advanced glass tubes and weight analysis, contributed to a deeper understanding of gas properties.
Antoine Lavoisier and the Naming of Hydrogen (1783)
French chemist Antoine Lavoisier built upon Cavendish's findings. In 1783, he officially named the element "hydrogen," derived from the Greek words hydro (water) and genes (creator), confirming that hydrogen played a fundamental role in water formation. His work, published in Traité Élémentaire de Chimie, laid the foundation for modern chemistry.
Modern research (Nature Reviews Chemistry, 2021) highlights the complexities of Lavoisier and Cavendish's studies, including initial calculation errors and challenges in modeling hydrogen reactions in the 18th century.
Industrial Use and 19th-Century Advancements
The Industrial Revolution and Early Applications
During the 19th century, hydrogen became an essential industrial gas. One of its earliest large-scale applications was in ammonia production, which later became vital for agricultural fertilizers. The Haber-Bosch process, developed in the early 20th century, remains a crucial method for large-scale ammonia synthesis today.
Scientific developments during this period were documented in journals such as Journal of Catalysis and Industrial & Engineering Chemistry Research, showcasing advances in catalytic processes for hydrogen applications.
Hydrogen Fuel Cells and Aerospace Applications (1960-Present)
In the mid-20th century, hydrogen played a pivotal role in space exploration. NASA used hydrogen fuel cells in Apollo missions and Space Shuttle programs as a lightweight, high-efficiency power source. These fuel cells combined hydrogen and oxygen to produce electricity, enabling long-duration space missions.
According to research in the International Journal of Hydrogen Energy, NASA faced challenges in early hydrogen fuel cell designs, such as weight constraints and limited efficiency. Modern advancements have significantly improved fuel cell performance, making hydrogen a key player in sustainable energy.
Hydrogen in the 21st Century: Challenges and Opportunities
Green Hydrogen and Clean Energy Transition
With the rise of climate concerns, green hydrogen—produced through water electrolysis using renewable energy sources—has gained global attention. Unlike hydrogen derived from fossil fuels, green hydrogen offers a zero-emission alternative for industries such as transportation, power generation, and manufacturing.
A study published in Nature Communications highlights key challenges in scaling green hydrogen production, including high energy costs and expensive infrastructure. Innovations such as graphene-based catalysts and new hydrogen storage solutions (e.g., methanol-based carriers) are helping address these barriers.
Innovations in Hydrogen Storage and Distribution
Recent breakthroughs in nanotechnology and material science have led to advanced hydrogen storage methods, including:
- Metal hydrides for compact storage.
- Cryogenic liquid hydrogen to improve transportation efficiency.
- High-pressure tanks for fuel cell vehicles.
Research published in Advanced Materials (2021) explores the use of nano-composites and advanced alloys to enhance hydrogen storage density, reducing costs and increasing feasibility for widespread use.
The Future of Hydrogen: Next Steps in Research and Development
Hydrogen as a Sustainable Fuel
With growing interest in hydrogen-powered transportation, major automakers such as Toyota and Hyundai are investing in fuel cell vehicles. Studies in the Journal of Power Sources and International Journal of Hydrogen Energy discuss advancements in fuel cell efficiency, cost reduction, and alternative hydrogen production methods.
Global Hydrogen Economy and Policy Developments
Countries worldwide are implementing policies to accelerate hydrogen adoption:
- European Union's Hydrogen Strategy (2020) aims to install 40 GW of electrolyzer capacity by 2030.
- Japan's Hydrogen Roadmap includes investments in fuel cell technology and hydrogen imports.
- United States Department of Energy (DOE) funds research on next-generation hydrogen fuel cells and distribution networks.
Environmental and Economic Considerations
Hydrogen's potential as a clean energy carrier aligns with net-zero emission goals. However, cost remains a significant factor. Reducing production expenses through scalable renewable energy projects and advanced electrolysis technologies will determine hydrogen's long-term viability.
The Application of Hydrogen Gas in Rockets as a Fuel Source
Introduction
With the increasing need for efficient and environmentally friendly energy sources, hydrogen has emerged as a critical fuel in aerospace and space exploration. Hydrogen is widely recognized for its high energy density, clean combustion, and capability to power liquid rocket engines used in space missions. This article explores hydrogen's role in rocket propulsion, its advantages, challenges, and future prospects in the aerospace industry.
Hydrogen as a Clean and High-Energy Fuel
Hydrogen is one of the most energy-dense fuels available, producing more energy per unit mass than any other chemical fuel. Unlike traditional fossil fuels, hydrogen combustion produces only water vapor, eliminating greenhouse gas emissions.
Production of Hydrogen for Rocket Fuel
Hydrogen can be produced through various methods, including:
- Electrolysis of water, using renewable energy sources such as solar and wind.
- Steam methane reforming (SMR), which is currently the most common method but involves CO2 emissions.
- Cryogenic distillation, which produces ultra-pure liquid hydrogen (LH2) suitable for aerospace applications.
Given the push toward sustainable energy, green hydrogen (produced via electrolysis using renewable energy) is gaining attention as a viable long-term solution for space exploration.
Hydrogen in Rocket Propulsion
How Hydrogen Fuels Rockets
Liquid hydrogen (LH2) is typically used in combination with liquid oxygen (LOX) as an oxidizer to create high-energy combustion. This mixture powers some of the most advanced rocket engines, such as:
- NASA's Space Launch System (SLS)
- SpaceX's Starship (under development for deep-space missions)
- Ariane 5 and 6 rockets (European Space Agency)
The LH2-LOX combination is favored due to its high specific impulse, meaning it provides superior thrust per unit of propellant compared to traditional kerosene-based fuels.
The Function of Hydrogen in Rocket Engines
In a liquid rocket engine, two main components are used:
- Fuel (LH2): Stored at extremely low temperatures (~ -253°C).
- Oxidizer (LOX): Required for combustion in space, where oxygen is not naturally available.
The fuel and oxidizer are pumped into a combustion chamber, where they ignite under extreme pressure, generating a powerful exhaust that propels the rocket forward.
Challenges in Using Hydrogen as Rocket Fuel
While hydrogen is an ideal rocket fuel, it presents several challenges:
Storage and Handling Issues
- Cryogenic storage: Hydrogen must be stored at ultra-low temperatures (-253°C), requiring advanced insulation and cooling systems.
- Leak risk: Hydrogen molecules are extremely small, making them prone to escaping through containment materials.
High Production and Infrastructure Costs
- The production, liquefaction, and storage of hydrogen require expensive infrastructure.
- Cryogenic fueling stations and specialized storage tanks add to the overall mission cost.
Efficiency and Weight Considerations
- Hydrogen has a low density, requiring large storage tanks, which increases the mass and complexity of rocket designs.
- Turbopumps and injectors must operate under extreme conditions to handle the cryogenic properties of hydrogen fuel.
Despite these challenges, advancements in fuel storage, insulation materials, and cryogenic engineering are continuously improving the feasibility of hydrogen as the primary fuel for next-generation rockets.
Advantages of Hydrogen in Aerospace Applications
Despite its challenges, hydrogen remains one of the best propellants for space missions due to:
- High specific impulse, meaning greater efficiency and longer flight durations.
- Clean energy, producing only water as a byproduct.
- Ideal for deep-space missions, as hydrogen can be extracted from lunar or Martian water ice for refueling future spacecraft.
Future of Hydrogen in Rocket Propulsion
Hydrogen and Reusable Rockets
Companies like SpaceX, Blue Origin, and NASA are investing in reusable launch systems, where hydrogen plays a crucial role in lowering costs and enabling rapid reusability.
Interplanetary Exploration
Hydrogen is also being studied for Mars missions and deep-space travel, with plans to use in-situ resource utilization (ISRU) to extract hydrogen from planetary ice deposits.
Advancements in Storage and Efficiency
New technologies, including metal hydride storage, graphene-based insulation, and supercooled hydrogen, are being developed to enhance hydrogen fuel efficiency and reduce weight constraints.
Conclusion
Hydrogen continues to be a critical component in modern rocket propulsion, enabling high-efficiency, clean-energy space travel. While challenges such as cryogenic storage and infrastructure costs exist, ongoing research and technological advancements are positioning hydrogen as the fuel of the future for deep-space exploration and beyond.
Hydrogen in the Food Industry
Various gases play essential roles in the food industry, including carbon dioxide, oxygen, nitrogen, hydrogen, and argon. Below is a brief overview of each gas's application:
Carbon Dioxide (CO2)
CO2 is the primary component in the production of carbonated beverages. Additionally, it acts as a cooling agent, helping companies freeze and preserve food products such as fish, meat, and beverages. This preservation method helps maintain flavor and texture while reducing the need for preservatives. Another advantage of CO2 is its ability to eliminate pests that attack vegetables, grains, and fruits without being toxic to humans.
Oxygen (O2)
Oxygen is used as an oxidizing agent to maintain the red color of meat. It also enhances respiration in plants and other food products, prolonging the freshness of vegetables.
Nitrogen (N2)
Nitrogen is commonly used to create an inert atmosphere in food packaging. By removing oxygen, microbial growth is minimized. Additionally, nitrogen aids in rapid cooling processes.
Hydrogen (H2)
Hydrogen is crucial in the hydrogenation process, which prevents the oxidation of oils and extends their shelf life. Hydrogenated oils have a higher smoke point, making them more suitable for frying. Additionally, hydrogen plays a role in controlled-atmosphere packaging (CAP) to preserve the freshness of certain perishable foods.
Argon (Ar)
Argon helps preserve food flavor by inhibiting respiratory enzymes.
What is Hydrogenation?
Hydrogenation is a chemical reaction between molecular hydrogen and an unsaturated compound, such as an alkene or alkyne, in the presence of a metal catalyst like rhodium, platinum, palladium, or nickel. By adding hydrogen to double or triple bonds, hydrogenation alters the molecular structure, effectively reducing the number of unsaturated bonds.
The hydrogenation of vegetable oils is an essential process in the food industry for producing semi-solid fats. During this reaction, hydrogen atoms are added to the carbon-carbon double bonds in unsaturated fatty acids, converting them into single bonds. This process turns unsaturated oils into saturated fats. In commercial applications, the degree of hydrogenation is carefully controlled to produce oils with the desired consistency. Hydrogenation is widely used in the food, petrochemical, and pharmaceutical industries.
Types of Hydrogenation
Hydrogenation reactions are classified into two types: full hydrogenation and partial hydrogenation.
Full Hydrogenation
In full hydrogenation, all double or triple bonds within a molecule are converted into single bonds by adding hydrogen. This process is commonly used in the petrochemical industry to transform alkenes and alkynes into more stable, less reactive alkanes. Fully hydrogenated oils do not produce trans fats, making them a healthier alternative to partially hydrogenated oils.
Partial Hydrogenation
In partial hydrogenation, only some of the double or triple bonds are converted, while others remain unchanged. This process is widely applied in the food industry to turn liquid vegetable oils into semi-solid fats like margarine. However, partial hydrogenation can lead to the formation of trans fats, which are harmful to health. Trans fats raise cholesterol levels, harden arteries, and inhibit the production of cyclooxygenase, an enzyme responsible for regulating blood vessel dilation and circulation, increasing the risk of heart disease.
Health Effects of Hydrogenation
Hydrogenated fats have been widely used due to their ability to improve food texture, extend shelf life, and enhance stability. However, growing concerns over the health risks associated with trans fats have led to regulations and industry shifts toward healthier alternatives. Studies from the American Heart Association and the World Health Organization (WHO) suggest that trans fats significantly increase the risk of cardiovascular disease, prompting many countries to ban or limit their use in processed foods.
Alternatives to Hydrogenation
With growing concerns over trans fats, researchers and food manufacturers are developing alternatives to traditional hydrogenation methods, including:
- Interesterification: A process that rearranges fatty acids in oils without creating trans fats, producing a similar texture to hydrogenated fats.
- Blending Oils: Combining different oils with varying saturation levels to achieve the desired consistency without hydrogenation.
- Enzymatic Modification: Using enzymes to modify fat structure naturally, enhancing stability and shelf life.
Applications of Hydrogenation in the Food Industry
The most common use of hydrogenation is in the production of hydrogenated vegetable oils. Vegetable oils consist of mono- and polyunsaturated fats. When unsaturated fats undergo hydrogenation, they transform into saturated fats with higher melting points. As a result, the final product is either semi-solid or solid at room temperature.
One of the most widespread applications of hydrogenation is converting liquid vegetable oils into semi-solid fats like margarine. Vegetable oils primarily contain unsaturated fats, which have one or more double bonds in their fatty acid chains. At room temperature, these oils remain liquid. Hydrogenation eliminates these double bonds, solidifying the oil into a semi-solid or solid fat.
How is Hydrogenation Performed in the Food Industry?
At the beginning of the hydrogenation process, vegetable oil is heated to a high temperature before hydrogen gas is injected. To accelerate the reaction, this process occurs in the presence of a metal catalyst, such as nickel.
During hydrogenation, double or triple carbon-carbon bonds in unsaturated fats break down, allowing hydrogen atoms to form single bonds with carbon atoms. This transformation results in saturated fats, which are generally solid at room temperature.
This process is widely used in margarine production. Hydrogenated margarine has a higher melting point and a longer shelf life compared to non-hydrogenated vegetable oils. Depending on the degree of hydrogenation, the final product can range from soft margarine to solid butter substitutes.
Where Can Hydrogen Gas Be Sourced?
Companies specializing in industrial and laboratory-grade hydrogen gas supply, such as Ardistan Gas Company, provide hydrogen in various volumes tailored to customer needs.
Sources & Further Reading:
- Nature Communications: Challenges in Large-Scale Green Hydrogen Production
- International Journal of Hydrogen Energy: Fuel Cells in Space Applications
- Advanced Materials: Nanotechnology for Hydrogen Storage
- Journal of Power Sources: Hydrogen Fuel Cell Vehicles
- European Commission - Hydrogen Strategy: https://ec.europa.eu
- U.S. Department of Energy - Hydrogen Research: https://www.energy.gov
- NASA - Liquid Hydrogen Fuel Technology: https://www.nasa.gov
- International Journal of Hydrogen Energy: Advances in Hydrogen Storage
- SpaceX & Blue Origin Reports: Developments in Reusable Hydrogen Rockets
- European Space Agency (ESA) - Hydrogen in Ariane Launchers: https://www.esa.int
- Cryogenic Engineering & Hydrogen Storage Research: https://www.sciencedirect.com
- https://cryospain.com/main-food-gases
- https://www.azom.com/article.aspx?ArticleID=16233
- https://mhcc.pressbooks.pub/chemfoodcooking/chapter/hydrogenation-and-oxidation
- https://testbook.com/chemistry/what-is-hydrogenation
- https://www.vaia.com/en-us/textbooks/chemistry/organic-and-biochemistry-for-today-8-edition
- https://www.yatlina.com/notebook/what-is-co2-extraction
- https://www.toptiontech.com/info/what-is-supercritical-co2-extraction
- https://gizmodo.com/what-is-hydrogenation-and-why-is-it-in-all-your-food-1718633937
- American Heart Association on Trans Fats
Related Articles
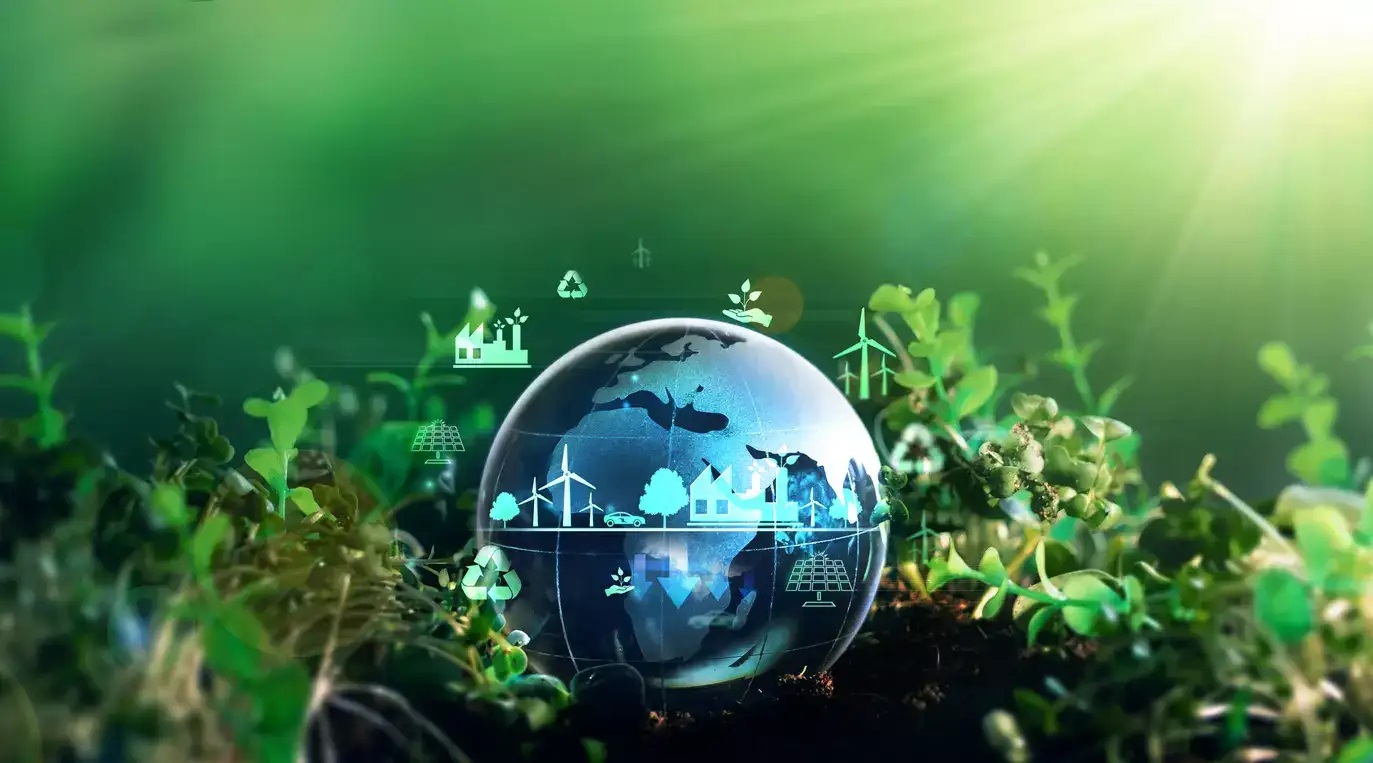
Hydrogen and Global Policies: Challenges and Opportunities
Hydrogen, the most abundant element in the universe, has long been used in industrial processes and energy production. However, in recent decades, its role in the global transition to sustainable energy systems has become more prominent.
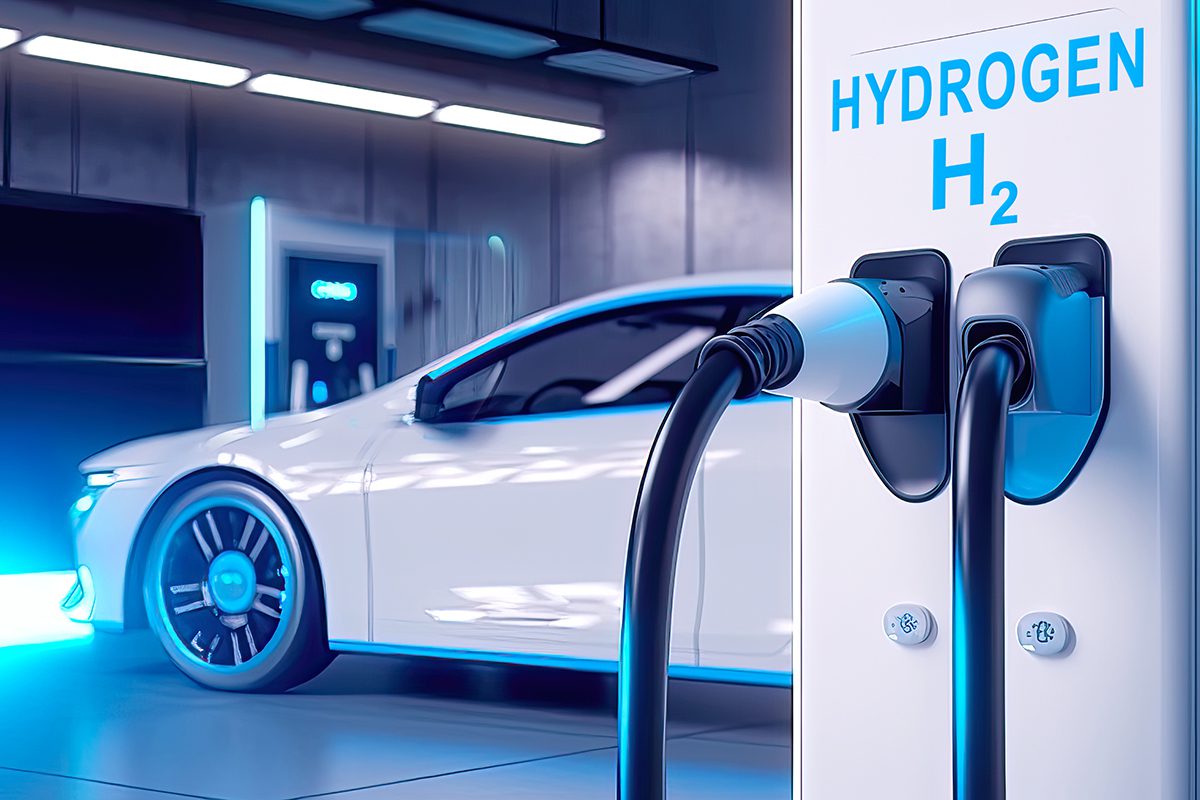
Introduction to Hydrogen Vehicles and How They Work
Since the invention of the first automobiles, vehicles have evolved into highly advanced machines.
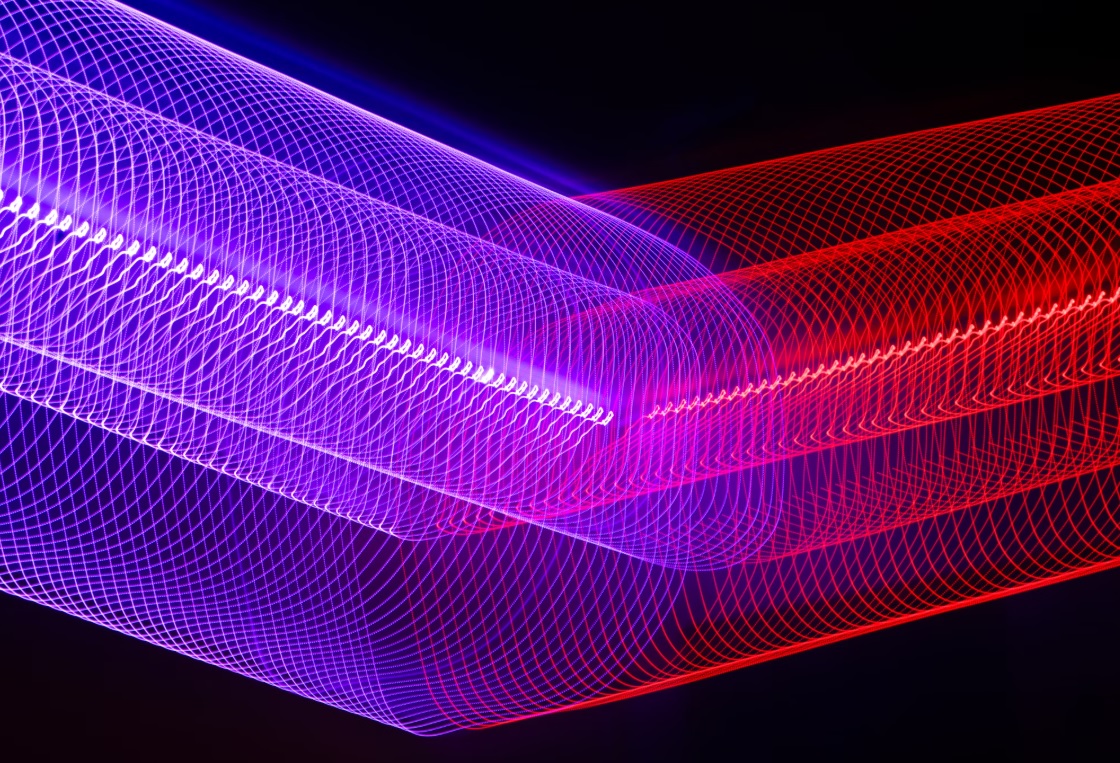
The Use of Hydrogen in Optical Fibers
Optical fibers are among the most innovative and essential technologies in modern communications and telecommunications. As advancements continue across various fields, one of the complex yet highly practical methods for improving optical fiber performance is the integration of hydrogen. Hydrogen plays a crucial role in enhancing the optical, mechanical, and chemical properties of optical fibers, making them more efficient for high-speed data transmission and long-distance communication.